Stockton’s New Atomic Force Microscope Will Allow Researchers to Resolve Atoms
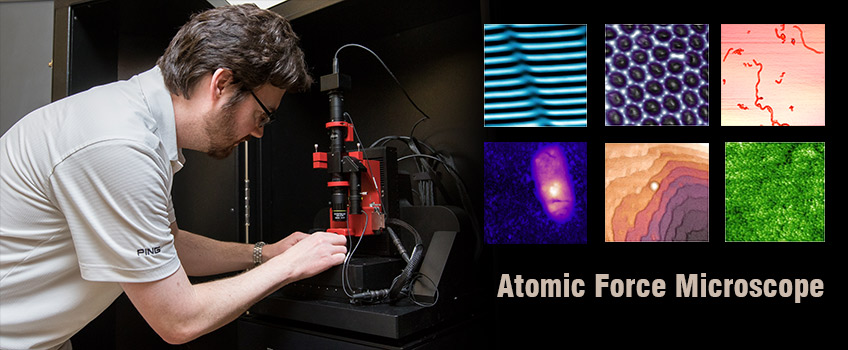
For Immediate Release; View photos on Flickr
Contact: Susan Allen
News and Media Relations
Galloway, N.J. 08205
Susan.Allen@stockton.edu
(609) 652-4790
www.stockton.edu/media
Galloway, N.J. – Stockton University students and faculty can create images of atoms and map the surface structure of materials using a new Atomic Force Microscope (AFM), advancing the university’s research into a realm once invisible.
“Stockton’s AFM is about 2,000 times more powerful than the best optical microscopes and it has the ability to resolve atoms,” explained Jason Shulman, assistant professor of Physics.
Students studying nanoscience, the study of material structure on the scale of nanometers, will be able to discover properties of materials that can only be seen with an AFM. Carbon nanotubes, strong tube-like structures made up of carbon atoms, are used in the medical industry for drug delivery and can also be used as electrical conductors. An AFM can help to characterize the structure and properties of a nanotube.
The AFM was purchased with funding from a $6.4 million Equipment Leasing Fund (ELF) state grant awarded to the School of Natural Sciences and Mathematics in 2013, which purchased most of the current cutting-edge scientific equipment housed in the Unified Science Center.
Stockton grants almost 22 percent of all the science and mathematics undergraduate degrees among New Jersey's senior state colleges and universities. Stockton’s Academic Quad expansion will include a $33.2 million Unified Science Center 2, where the microscope will eventually be housed.
Using an atomic force microscope at the undergraduate level prepares students for graduate research and their future careers.
The new microscope, manufactured by NanoMagnetics Instruments, looks somewhat similar to a traditional optical microscope, but its functionality and capabilities are vastly different.
Instead of looking through an eyepiece lens at samples illuminated with light, the AFM generates a computer image after a needle systematically scans the sample and sends the information to be analyzed by software.
“The needle deflects upward when the surface rises and downward in valleys. A computer registers the amount of deflection. The end result is a topographical image of the surface,” Shulman explained.
The resulting image is color coded to define the surface structure and to reflect the variations in height, characterizing textures that cannot be seen by the eye.
Optical microscopes can only magnify objects to a size that is on the order of a wavelength of light. With an AFM, light is no longer a limitation, allowing magnification on the scale of nanometers (one billionth of a meter).
The AFM is mounted on a vibration isolation platform, which will negate any movements caused by sound vibrations or movement in the vicinity, and is housed within an acoustic, thermal and vibration isolation cabinet.
When imaging small samples, the slightest vibrations cause errors. Dust also becomes magnified.
“Watch out for the dust particles,” warns Shulman, as he places a sample under the needle.
A speck of dust can be enlarged to the size of a dime in an AFM image.
In addition to topographical measurements, the AFM can also visualize the electronic, magnetic and mechanical structure of surfaces, and more, helping physics students to better understand small scale physics.